AUV Technology
Annotated list with links to reports or papers.

AUV Technology
Introduction and scope
It is not the intention that this section covers all of the sub-systems technologies that are to be found in modern AUVs. Rather, the aim is to cover those sub-system technologies that face greater, or indeed unique, challenges in the Polar Regions. Within each sub-system, the component technologies likely to be affected are described together with examples of successful deployments and implementations. Scattered throughout are examples of where AUV teams have faced difficulties with technologies and particular implementations. As we take the definition of technology to be, "Technology is an enabling package of knowledge, devices, systems, processes and other technologies, created for a specific purpose" we include discussion of risk management systems and processes within this section.
The sub-system technology challenges are outlined below. 'Read more' links take you to pages that showcase some of the latest research in those areas.
Navigation
Navigation is a broad term; within our scope there are two main aspects where operating in the Polar Regions give rise to additional and unique challenges.
• Positioning: obtaining an estimate of the true position of the AUV, where an AUV sub-system may take data from several external sources, e.g. GPS satellites when on the surface or a network of acoustic beacons when sub-surface, or may match its current position to a previously known location, e.g. using terrain navigation, or may have its position estimated and updated from a remote location, e.g. a surface ship using USBL. In the Polar Regions sea or shelf ice may mean an AUV cannot surface for GPS position fixes and an operator may face the substantial challenges of installing and using a long range acoustic navigation system, for example as envisaged by the 2006 Anchor Workshop.
• Dead Reckoning: using speed (ideally over ground) and heading (ideally referenced to true North) to obtain an estimate of current position in the absence of position fixes and, in post-processing, to obtain an improved estimate of the track from some form of optimum interpolation between position fixes. Measuring heading poses particular problems in the Polar Regions, with the proximity of the Magnetic Poles affecting magnetic techniques and gyroscopic techniques that depend on the rotation rate of the earth affected by an error term that grows as the secant (1/cosine) of latitude.
Read more on recent concepts in AUV Navigation in the Polar Regions ...
Homing and Guidance
Only in special circumstances, e.g. where an AUV is to locate a subsea docking station, does a civil AUV operating in ice-free waters have to include a homing and guidance system. In Polar Regions an effective homing and guidance system is necessary for several modes of operation, including:
• Reaching the end of a mission (or a monitoring waypoint) at an ice camp that may be drifting, where the exact position could not be known and programmed as a fixed waypoint when the mission started.
• Needing open water at the end of a mission for recovery to a ship at a location prone to sea ice, where the ice conditions at the recovery place and time might be uncertain at the time of launch, e.g. due to changes in wind force and direction.
Read more on recent concepts in Homing and Guidance solutions ...
Collision Avoidance
The under ice environment can be a complex 3D world of obstacles that may scatter or specularly reflect sound. The challenges for collision avoidance hardware (inevitably sonar) are difficult enough, but the real challenge comes in the real-time interpretation of what may be sparse data on an environment where preconceived notions and ideas may be quite wrong.
Seabed features such as strudel scour and under ice features such as ice keels tens of metres deep under sea ice to chasms hundreds of metres high under ice shelves and glaciers as well as scattered icebergs pose multiple difficulties for AUV collision avoidance systems not seen in open water. Practical solutions have ranged from collections of sonars with single or several beams to systems with tens of beams aiming to cover the full four-pi steradian solid angle.
Read more on recent concepts in Collision Avoidance ...
Communications and Telemetry
Regular surfacing for satellite or terrestrial radio communications and telemetry is more difficult and risky in a region with sea ice compared with open water, and indeed may be impossible when under fast ice or ii an area with 10/10ths ice cover. Current techniques for radio communications are not suitable at all for under ice shelf environments, but an innovative systems-approach may hold some promise.
Acoustic communications and telemetry in the Polar Regions may be strongly influenced by strong vertical gradients of sound speed especially if the upper layers comprise cold, fresh melt water. Physical obstacles such as ice keels that scatter sound, or give rise to shadow zones may also affect acoustic communications range and reliability.
Communications and telemetry play an important role not only in the regular conduct of missions but also in locating a missing vehicle, especially when it may be under ice, and special adaptations may be needed to ensure an adequate communications range under those circumstances.
Read more on recent concepts in Communications and Telemetry ...
Buoyancy Control
Achieving an appropriate buoyancy can be a difficult task for an AUV operating in the Polar Regions, where large differences in density (temperature, salinity) can occur either in the vertical or the horizontal.
Some vehicles adopt a strategy of determining a suitable static buoyancy before launch and rely on the forward motion of the AUV and the dive planes and the depth-control algorithms to maintain the required depth despite changes in water density. This can come at the cost of additional energy consumption from the vehicle body taking up an angle of attack that incurs additional drag. This has been the approach with the Autosub series of AUVs on under ice missions.
Other vehicles have used an active buoyancy (or ballast) control system, most notably for an under ice AUV - that used on the ISE Theseus for its Arctic cable-laying missions. Not only did the system have to cope with water density changes (estimated to result in a buoyancy change of 400 lbs) but also the change in displacement as the fiber optic cable was deployed onto the seabed. An arrangement of pumps and valves let water in, or pumped water out, of toroidal tanks in the free-flooding bow and tail while maintaining trim.
Read more on recent concepts in Buoyancy Control ...
Energy Storage
A good knowledge of the energy system performance in cold temperatures should be a prerequisite for effective mission planning. A realistic model of the energy system performance with load and temperature can help. In temperate regions it can be a problem for an AUV energy system to avoid reaching to high a temperature, and the hardware design may need to incorporate cooling devices. In Polar Regions the AUV is likely to face the problem of conserving self-generated heat to maintain a high enough working temperature for efficient energy conversion. Solutions include choice of energy source technology, insulation, and pre-heating the energy source before deployment.
Read more on recent concepts in Energy Storage ...
Instruments, Sensors and Samplers
There may be some missions for which an instrument or sensor specific to the Polar Regions will be needed, but more often, an instrument or sensor commonly used in temperate regions may suffice, although modifications may be needed. Some modifications that may initially seem trivial, such as turning a swath bathymetry transducer array through 180˚ to image the under side of ice rather than the seabed, but end up exposing hidden complexities and assumptions that may cause unforeseen problems.
Icing on deck may cause problems for some sensors and samplers that have internal water-containing cavities or tubes, low temperature may affect calibration, for instance in the backscatter measurement from scientific echo sounders. Supercooled frazil ice may result in false measurements, for example false echoes from an echo sounder, or it may coat or clog temperature and conductivity sensor surfaces.
AUV operations in Polar Regions offer great scope for innovative underway sensors, such as for measure the amount of algae growing on the underside of sea ice.
Read more on recent concepts in Instruments, Sensors and Samplers ...
Risk Management: Systems and Processes
Risk Management is included in this section on AUV Technology as an "enabling package of knowledge". While many, if not most, AUV operators practiced informal methods of risk management, perhaps described as "good engineering practice", and some groups thought about replicate and redundant sub-systems or sensors, it was not until after the report of the investigation into the loss of the Autosub2 AUV under the Fimbul Ice Shelf in 2005 that ideas on formal risk management systems tuned to the needs of AUVs were published in the peer reviewed literature.
Some of the ideas were controversial, several researchers considered that only through the methods of classical probability, testing many of the same sub-system modules and obtaining reliability statistics, could the reliability of an AUV be assessed. Ideas centred on subjective (or personalist) probability and formal expert judgment were considered anathema by some. Nevertheless, a number of AUV groups operating vehicles in Polar Regions have taken up, and implemented, formal risk management strategies. Sume groups are using formal expert judgment to assess and improve the likely outcomes, a key example being the team from Natural Resources Canada, DRDC and ISE, guided by the University of Southampton, with the Explorer AUV's Arctic missions.
Read more on recent concepts in Risk Management: Systems and Processes...
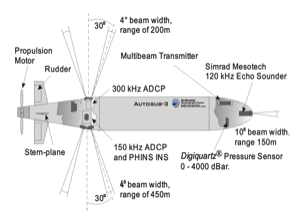
Diagram of the Autosub3 AUV as configured for its missions beneath Pine Island Glacier in 2009, showing the three sonars used for collision avoidance: Upward-looking four-beam 300kHz ADCP, downward-looking 150kHz ADCP and a 120kHz single beam forward-look instrument. From McPhail et al. (2009).
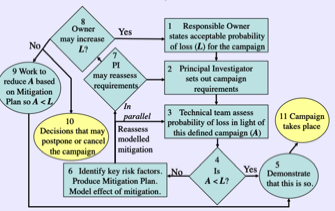
Flow chart showing an early version of a Risk Management process proposed for use with under ice Autosub operations after 2006. From the chapter by Griffiths and Trembanis in the Proceedings of the 2006 AUV Technology Masterclass.
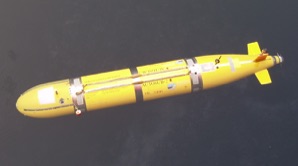
The Autosub2 AUV fitted with upward-looking swath bathymetric sonar on missions off North East Greenland in August 2004. The transducers are beneath the white acoustic windows.
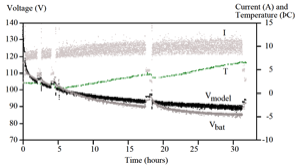
Graph showing temperature rise for insulated manganese alkaline batteries within Autosub2 in under ice missions in 2001 together with predicted and actual battery voltage. From Stevenson et al. (2002).
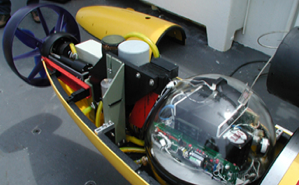
The rear section of the APOGEE (Autonomous Polar Geophysical Explorer) AUV from Woods Hole, with its acoustic communications and telemetry transducers visible astern of the glass sphere. Image from WHOI.
Sprint-Nav from Sonardyne incorporates the company's Sprint INS, based on three ring laser gyros, with its Syrix Doppler Velocity log in a single package capable of dead reckoning with a cumulative error of less than 0.04% of distance travelled in a typical survey pattern. This solution has been chosen for the Autosub Under Ice 2K AUV currently in development. Image from Sonardyne.
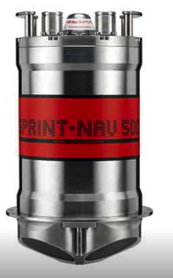
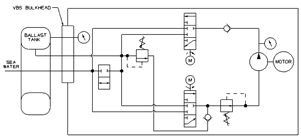
Outline of the large Penn State/ARL Seahorse AUV Variable Buoyancy System. The fore and aft reservoirs were rubber tubes into which water could be pumped or released. Able to operate down to depths of 350 m the positive displacement pumps could move 9 kg of water a minute. From Tangirala and Dzielski (2007).