Recent concepts, developments and practical experience
Concept of Under-Ice navigation Buoys
The concept of a network of free-drifting buoys to aid under-ice navigation of AUVs has been explored by Norgren et al. (2020). Each buoy would carry an above-water Global Navigation and Satellite System (GNSS) to obtain position fixes and a two-way underwater acoustic modem (e.g. the WHOI Micromodem) for bidirectional communication with one or more AUVs. Communication to a support ship would be via short-range VHF radio but also via Iridium satellites. Buoys would be deployed along the planned route of the vehicle(s), either as a transect or in an operating area, for example in support of a grid survey. The authors consider that, "most important advantage of deploying intelligent buoys when performing AUV missions under the ice is bounding the navigational error throughout the AUV mission".
In this collaboration between the Norwegian University of Science and Technology, the University Centre in Svalbard, the Norwegian Defence Research Establishment and Kongsberg Underwater Technology simulations have centred on the capabilities of the REMUS 100 AUV aided by the additional horizontal position estimate from the buoys into a common Kalman Filter.
Use of Extremely Low Frequency Electromagnetic Waves for under ice AUV localisation
Yoshida et al. (2020) have proposed and studied the use of ELF radio waves for AUV localisation especially in areas where acoustic propagation would prove difficult.
Terrain Aided Navigation for under ice AUV localisation
Liu et al. (2020), recognising the very low spatial resolution of bathymetric maps of the Arctic seafloor have simulated a particle-filter approach for implementing terrain-aided navigation.
Navigating an AUV across the Arctic basin with terrain-aided navigation and coarse bathymetric maps was the problem set out in Salavasidis et al. (2021). Thie motivating example is an over 3200 km mission between Svalbard in the Greenland Sea to Utqiagvik (Barrow), Alaska. Their approach combines the sparse bathymetry with a model of its uncertainty with a numerical model of Arctic Ocean currents and a detailed model of the errors of the heading sensor. Valuable conclusions include:
• Dead reckoning (DR) with a magnetic compass and water-track ADCP measurements may give basin-scale navigation errors, in their worst case example, "up to 2400 km".
• Using an north-seeking fibre-optic gyrocompass reduced the DR error, but the worst case error was unacceptable, at 420 km.
• The bathymetric map used was from IBCAO with a grid spacing of 0.5 km, recognising that large areas with no actual data have been interpolated. Their uncertainty model assigns a standard deviation to each grid cell based on the data source code for that cell.
• The NEMO numerical model simulated currents on a 3D grid with a spacing of 0.083˚.
• In an example of a path averaged over successful runs of their combined models the RMS error between the true and terrain-aided navigation path was 3 km, with a maximum of 39 km (config.5 suite) shown in the graphic opposite. Note that the chosen path avoided, as much as possible ,areas of high standard deviation in bathymetry.
Virtual environment to construct a navigation framework for an AUV on Arctic missions
Evaluating the likely performance of different navigation technology sub-system components in the Polar Regions can be logistically difficult and costly, and limited time in the field can lead to slow progress. Laboratory-based virtual environments therefore have a useful role in sub-system selection from providing estimates of likely performance under actual conditions.
Randeni et al. (2020) constructed a physics-based virtual environment simulator including the following capabilities:
• An environmental simulator for the ocean, Arctic ice and ice motion, and the ocean acoustics environment and propagation.
• A vehicle simulator including vehicle dynamics and navigation sensors.
• 'Top-side' hardware and acoustics communications simulator using either a software-only version or a Hardware-in-the-Loop version based on Netsim.
• Interfaces between real sub-system components were also simulated as it was considered, "critical to test the entire software pipeline in order to succeed by largely seamlessly switching from simulation to runtime in-field operations". Hundreds of hours of mission simulations helped identify failure modes, pointed to corrections needed and allowed those corrections to be tested.
Determining the AUV position through combined EM and acoustic methods
In a short abstract Shaidurov et al. (2020) suggest a method for determining the position of an AUV "without surfacing (including under Arctic pack ice" based on the interaction of electromagnetic and acoustic waves "in the skin layer of sea water". This parametric effect was confirmed in a laboratory tank, where the difference frequency of the electromagnetic and acoustic waves was received underwater.
Minimising external inputs needed to navigate a glider in the Marginal Ice Zone
In a wide ranging and thought-provoking article Duguid and Camilli (2021) explore several methods by which a glider operating near the ice edge can navigate safely with satellite updates of order one a day. The topics covered, all informed by real-time environmental state-estimation, include:
• Intelligent power management, leading to energy savings, e.g. through only using essential sensors when on transits.
• Hybrid buoyancy engine and propeller propulsion together with an adaptive velocity control using a multi-factor objective optimisation approach. The propeller option allows the glider to make headway even in the presence of adverse currents.
• Dynamic depth band selection, not just the saw-tooth profile constrained by a buoyancy engine alone. This capability can benefit scientific sampling and improve energy efficiency for shallow profiling with a deep glider.
• Sea-ice sensing using a mechanical scanning sonar not only provides an estimate of the draft and distribution of ice overhead, but an analysis of the Doppler shift is expected to give estimates of wave height, period and direction. These data on the surface environment can aid navigation and surfacing decisions by the glider's controller as well as being telemetered to shore as summaries of ice conditions.
Ice avoidance route planning for long-range AUV missions
Fox et al. (2022), in a paper that has been made available while in review, propose a set of methods to achieve three main objectives for long range AUV missions in areas where sea ice may be present:
• Limitations of present algorithms that rely on monthly, gridded maps of ice presence and type can be improved upon using their proposed combination of Dijkstra's algorithm and Newton's method. Dijkstra's algorithm finds the shortest overall journey time taking into account sets of constraints.
• A user-set risk avoidance threshold allows for routes that may be more time efficient but carry a greater risk, or vice versa.
• Surface currents are accounted for, using a model that has been constrained by observations from the Biogeochemical Southern Ocean State Estimate.
Navigation of AUVs beneath moving sea ice
Barker and Whitcomb (2021) provide a wide-ranging review of published work on the problem of ice-relative navigation of AUVs, whether the ice is stationary or moving. Their proposed method, which they have tested with simulations, uses two or more satellite navigation beacons on the ice surface together with the navigation sensors on the AUV, e.g. upward DVL, and support ship in an extended Kalman Filter. One area of uncertainty the authors highlight is that of the actual path of sound between source and receiver - they have assumed straight paths - while acknowledging that refraction could occur and would add to distance sensor noise.
From Norgren et al. 2020, the AUV at the centre receives position updates via acoustic modems from the buoys shown with velocities v1 and v2, but the buoy with v3, intended to cover the later track is currently out of range.
Navigation

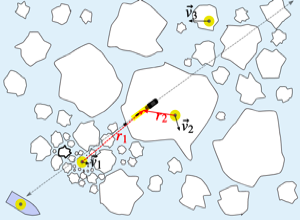
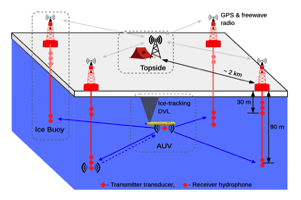
From Randeni et al. (2020), an outline schematic of the key components of an integrated communications and navigation network to provide position updates to an AUV operating beneath ice.
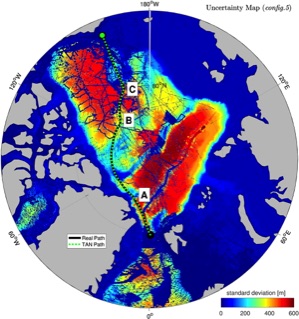
From Salavasidis et al. (2021) a map showing the true and terrain-aided navigation track of an AUV across the Arctic Ocean basin. The colour infil is the standard deviation of the bathymetry. Maximum RMS deviation for this track was 39 km.
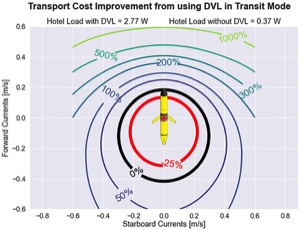
From Duguid and Camilli (2021), a diagram showing the improvement in transport cost by using the DVL on transits to avoid along and across track currents. If both are small, that is, within the black circle, there is no advantage to using the DVL. Substantial benefits, over 200%, are possible if head currents are avoided.